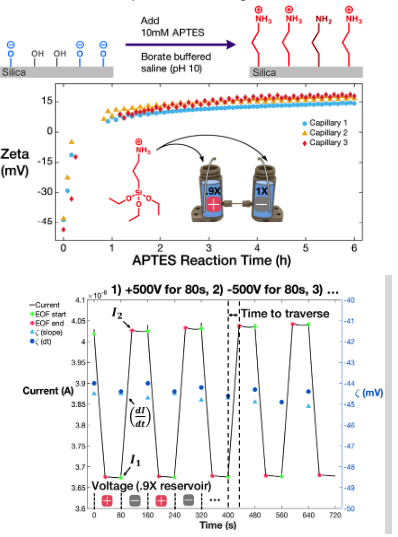
The ability to monitor chemical surface reactions precisely and in real-time can provide fundamental insights to help us create highly relevant and complex nanoscale technologies (e.g. cell surface engineering, surface-anchored biosensors, 3D nanostructuring, etc.). The kinetics of self-assembled monolayer formations have been reported for several types of reactive groups (trimethoxysilanes, thiols, etc.). However, there is still a need for affordable, cross-compatible, in-situ surface analysis systems with high temporal resolution to monitor reactivity and stability of self-assembled monolayers in a variety of conditions. Therefore, our goal is to develop a fully autonomous platform (i.e. no user interaction) to monitor surfaces in real-time after exposure to a library of surface-reactive compounds.
Thus far, in the Surface Chemistry Division of the Pennathur Lab we have demonstrated the use of electrokinetic nanofluidics to precisely quantify the physicochemical composition of surfaces in a controlled aqueous environment (temperature, pH, ionic strength, viscosity). Through monitoring current, we can measure electroosmotic flow (EOF) velocity in nanometer-width channels (nanochannels) in an applied electric field. We can then relate EOF velocity to the zeta potential, a net potential located after a layer of ions which forms along a surface to reduce charge imbalances (resulting from surface chemistry). For more detail, please see our section on Conductivity/Concentration at Nanoscale.
We have modified this frame-by-frame technique to now capture dynamic chemical processes. To do so, we apply a cyclic program to alternate voltage polarity at set time intervals, thereby actuating the flow of new fluid through the channel from left or right reservoirs at the start of each voltage cycle. We extract EOF velocity by adding a small (but measurable) difference in conductivity between reservoir solutions and monitoring the change in conductivity as new solution traverses the channel. The use of nanocapillaries ensures EOF measurements are unaffected by reservoir pressure differences, and the use of very short tubes allows us to reduce the amount of time required for fluid to traverse the channel, thereby increasing our temporal resolution for continuous zeta potential monitoring.